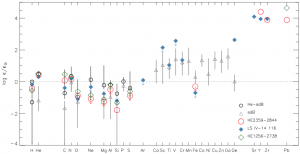
This figure shows chemical abundances for several stars relative to the Sun. The compositions for the unusually lead-rich stars are shown as a red circle, blue diamond, and green diamond.
Today in journal club, we discussed two papers.
We started with Schlichting et al. (2013), which looked at the size distribution of Kuiper belt objects (KBOs) to figure out how they formed and evolved over the history of our solar system.
Since, as the paper says, the Kuiper belt “is a remnant from the early solar system”, its size distribution depends on the accretion processes that gave rise to planets and also on the collision processes that affect many aspects of planetary formation and evolution.
KBOs range in size from unobservabley small to about a thousand kilometers (km) in radius (Pluto-sized), but the numbers of objects of a given size depend on how the objects formed and on the nature of the collisions among the objects (which can break up the smallest objects).
Schlichting and colleagues argue that the size distribution of KBOs suggests that smaller objects (less than about 30 km in radius) have been dominated by collisions, while bigger objects are much less affected by collisions. So the bigger objects may provide a glimpse into the early history of our solar system.
The other paper was Naslim N. et al. (2013), which announced the discovery of the most lead-rich stars ever found. The stars discussed in the paper are sub-dwarf stars — essentially very small, retired stars — and have thousands of times more lead in their atmospheres than our Sun.
Not only is the amount of lead surprising, it’s surprising that we can see the lead at all. Since lead is so much heavier than the hydrogen and helium that make up most of the stars’ atmospheres, we would expect the lead to settle out of the atmospheres, deep enough in the star that we couldn’t see it.
However, something has caused the lead in these stars to remain suspended in their atmospheres, but not other heavy elements. The authors suggest preferential radiative levitation (essentially some kind of interaction between the atoms and the stellar light) keeps the lead suspended but not other heavy elements.
- August 20th, 2013
- Posted in Journal Club
- Comments Off on Primordial Sizes of Kuiper Belt Objects — Schlichting+ (2013); Discovery of extremely lead-rich subdwarfs — Naslim N.+ (2013)
- August 15th, 2013
- Posted in Brian's publications
- Comments Off on “Time Really Flies on These Kepler Planets” — Irene Klotz
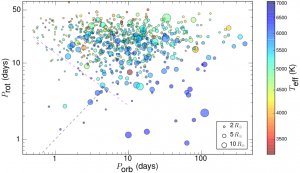
Figure 2 from McQuillan+ (2013) showing rotation periods of host stars (P_rot) vs. orbital periods of planetary candidates (P_orb). The size of the circle indicates the size of the planetary candidate, and the colors indicate stellar temperature.
In journal club today, we first discussed the recent tenth release of data from the third Sloan Digital Sky Survey (SDSS III). The survey basically takes a mammoth picture of the sky every night and provides a wealth of astronomical data that can be used to map the Milky Way, search for extrasolar planets, and solve the mystery of dark energy. According to the wikipedia, SDSS collects about 200 GB of data every night, so, over the last year, the survey has collected tens of terabytes of data.
We also talked about a recent paper by McQuillan, Mazeh, and Aigrain. These authors analyze the brightness variations of many stars observed by the Kepler mission that are orbited by planetary candidates.
Like the Sun, many stars have star spots, cooler and darker regions on their faces, and these spots rotate in and out of view as the stars rotate, just like those on the Sun. When the spots rotate into view, the star appears to darken slightly, and so you can use brightness variations to determine the star’s rotation rate. (Watching the motion of sun spots is how the Sun’s rotation rate was first determined.)
The authors suggest a correlation between the rotation periods of these host stars and the orbital periods of their planetary candidate companions: there is a dearth of planetary candidates with short orbital periods around stars with short rotation periods.
Our journal club group raised several questions about these results. Among them, we wondered whether there are any biases in the sample of studied stars that could account for the correlation. Also, could it be harder to find planetary candidates around faster rotating stars (faster rotating stars could exhibit brightness variations rapid enough that they confuse the Kepler transit search for planets) — could this idea account for the correlation?
However, if no underlying biases or sampling issues account for the correlation, then the results say something interesting about the connection between planets and stars. Why do planets close to their stars (i.e., with short periods) seem to prefer orbiting stars that rotate slowly?
- August 13th, 2013
- Posted in Journal Club
- Comments Off on SDSS III 10th release; “Stellar Rotation Periods of the Kepler Objects of Interest” — McQuillan+ (2013)
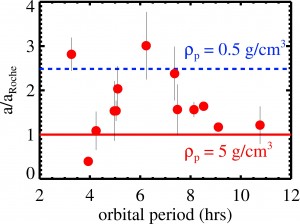
Figure 20 from Jackson et al. (2013) showing the distance from their host stars at which planets would be torn apart (solid red line).
Today I submitted a new paper for publication, “A Survey for Very Short-Period Planets in the Kepler Data.” Our new survey of data from the Kepler planet-hunting mission has revealed planetary candidates with orbital periods as short as three hours, so close to their host stars they are nearly skimming the stellar surface.
We used data from the Kepler mission, which finds planets using the transit method — by looking for their shadows as the planets pass between their host stars and the Earth. Since the planets are so far from the Earth, we can’t see them directly and instead only see the little dip in brightness of the host star as the planet passes in front of it.
Over the last few decades, astronomers have found a breath-taking menagerie of exotic planetary systems, and the candidate planets we found in this paper are no exception: more than 100 times closer to their host stars than the Earth is to the Sun, if these candidates turn out to be rocky planets, their surface are baking at nearly 5,000 degrees F (3000 K), producing giant lakes of molten rock.
In fact, these planets are so close to their host stars that they are on the verge of being torn apart by the stars’ gravity. The figure at left shows the orbital distances for these planets (red dots) relative to the distance at which they would be torn apart (shown by the red line). The blue line shows where they would have been torn apart if, instead of being rocky, they were more like hot gas giant planets.
We’ve still got some work to do to make sure these candidates are actually planets, but if confirmed, they would be some of the closest planets to their stars ever discovered, once again overturning what astronomers thought we knew about where planets can live and what they’re like.
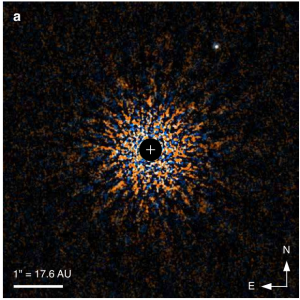
The false-color, infrared image of the GJ 504 system. The star (center) has been blocked out, and the planet appears a white blob at the upper right.
At today’s journal club, we discussed the recent paper Kuzuhara et al. (2013), which reports the discovery of an extrasolar planet with a mass of about 4 times Jupiter’s mass but in an orbit more distant from its sun than Neptune is from ours.
The image at left shows one of the discovery images, taken at infrared wavelengths. At these wavelengths, usually a planetary host star is shining much less brightly than at visible wavelengths, which makes it easier to see radiation from the planet. However, for the kind coronagraphic imaging here, the star still has to be blocked out to prevent the glare from masking the planet (and blocking out the star completely is hard to do, as evidenced by the orange and blue speckles in the image).
This planet is particularly interesting because it is the oldest planet (it’s at least 160 million years old) to be observed with this kind of direct imaging technique. Younger planets are usually hotter and emit more radiation, and so it’s easier to see them when they’re younger.
Although this system is one of the oldest directly imaged, it’s still very young compared to our solar system, which is about 4.5672 billion years old. 160 million years after our Sun formed (and the solar system got its start), the Earth and other planets had already formed, but the Earth was very different from the way it is today. For example, water may have already collected in the oceans, but our atmosphere was probably composed of carbon dioxide and water, with essentially no oxygen. Oxygen came later when life got started.
So studies like this one can allow us to peer back in time to figure out, among other things, what planets look like when they are very young and piece together to story of our solar system.
- July 31st, 2013
- Posted in Journal Club
- Comments Off on “Direct Imaging of a Cold Jovian Exoplanet in Orbit Around the Sun-Like Star GJ 504” — Kuzuhara+ (2013)
Today’s DTM Seminar speaker was Prof. Zhigang Peng from GA Tech‘s Earth and Atmospheric Sciences Dept. He talked about a kind of earthquake (he called “tremors”) that is triggered when the seismic waves from another larger earthquake pass by. The relationship between earthquake duration and strength (as measured by the seismic moment) for these tremor quakes is apparently much different than for normal earthquakes, suggesting they result from different geophysical mechanisms.
The part of the talk that stuck out most for me was when Prof. Peng played the “sound” of an earthquake, generated by speeding up the seismic vibrations from measured earthquakes. This “earthquake music” is available on his website, but I have posted one such recording below. Apparently, different kinds of earthquakes make different sounds, and so these recordings can be used to tease out information about the quakes.
Sound of the 2004 Parkfield CA earthquake
- July 30th, 2013
- Posted in DTM Seminar
- Comments Off on “Triggered Tectonic Tremor and Interaction of Large Earthquakes”
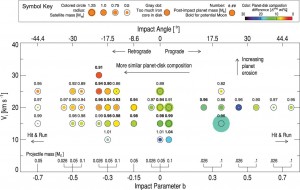
Figure 2 from Cuk & Stewart (2012) showing the range of model outcomes for different impact conditions.
In Friday’s DTM seminar, Sarah Stewart-Mukhopadhyay of Harvard gave a fascinating talk on the origin of the Moon and the effects of large impacts on the Earth’s volatile inventory.
Lots of evidence (geochemical and astronomical) say that the the Moon originated in a large impact between the proto-Earth and a roughly Mars-sized rogue planet (often called Theia). This explanation accounts for many of peculiarities of the Earth-Moon system, EXCEPT that geochemical data says that Earth and Moon probably formed out of the same material. Old models of the Moon’s formation showed that Moon should have formed mostly out of the impactor, Theia, which (like all other solar system planets) was probably compositionally distinct from the Earth. So the Moon SHOULDN’T be Earth’s compositional twin.
Instead, Stewart and Cuk suggested that the pre-impact Earth was spinning much faster than previously assumed, with a day that lasted about 2 hours, and so enough material from the proto-Earth could have been launched by the impact to make the Moon mostly out of the proto-Earth. Once the Moon formed, Stewart and Cuk suggested it could interact gravitationally with the Earth and the Sun to reduce the Earth-Moon angular momentum and bring it into line with what we see today, removing the one big objection to their fast-spinning proto-Earth idea.
In the last part of her talk, Stewart discussed what such large impacts might do to the gases trapped inside the young Earth. Contrary to popular thought, she suggested that these large impacts actually might NOT melt most of the Earth’s outermost layers, and so the Earth could retain in its interior much of the nebular gas that it was born with.
This idea could help explain geochemical data that suggest gas trapped in different parts of the Earth’s interior currently have different isotopic compositions and would mean that the Earth retains geochemical scars from its adolescence once thought completely erased by the violence of the early solar system.
- July 27th, 2013
- Posted in DTM Seminar
- Comments Off on “New Ideas about the Origin of the Earth and Moon”
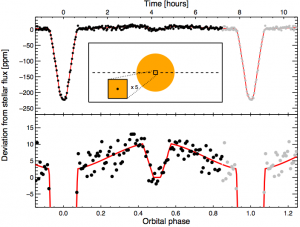
Figure 3 from Sanchis-Ojeda+ (2013) showing observations of the Kepler-78 b’s shadow as it passes in front of its host star.
I sat in on University of Maryland Astronomy’s colloquium, today given by Roberto Sanchis-Ojeda of the Kavli Institute at MIT. He discussed his recent paper announcing the discovery of Kepler-78 b, an Earth-sized planet with on orbital period of only 8.5-hours. In such a short orbital period, this planet is only 0.01 AU distant from its host star, almost 100 times closer to its star than the Earth and 40 times closer than Mercury are to our Sun.
Unfortunately, since the planet is so small, we don’t have a direct estimate of its mass, but so close to its sun, the planet’s day side is baking at a temperature probably greater than 1,500 K (2,200 degrees F), above the melting point of rock — The planet’s day side is probably covered by a lake of molten rock.
Kepler-78 b is one of a few very hot rocky planets discovered so far and the shortest-period transiting planet confirmed. CoRoT-7 b was the first one (discovered by the CoRoT mission), and Kepler-10 b was the first rocky planet discovered by the Kepler mission. The origins of these very hot rocky planets are unclear — they probably didn’t FORM so close to their host stars (although some have suggested rocky planets MAY form very close to their host stars). In any case, they represent yet another entirely unexpected but exquisitely interesting discovery in extrasolar astronomy.
- July 23rd, 2013
- Posted in UMD Seminar
- Comments Off on “Kepler-78b: Transits and occultations of an Earth-sized planet in an 8.5 hour orbit”
In journa
l club this morning, we discussed the recent paper by Deming et al. (2013), just accepted for publication by the Astrophysical Journal. In this study, the authors observed the planetary transits of HD 209458 b and XO-1 b using the Hubble Space Telescope in the near-infrared (about 1-1.6 microns in wavelength) to look for spectral features of water and other molecules. These features tell us whether water and other molecules are present in a planet’s atmosphere, how abundant those molecules are, and even where the atmosphere they are located. In this case, the authors saw spectral absorption from water molecules (see the left portion of the figure at left), but the amount of absorption (the depth of the absorption band) isn’t as large as it would be if the atmosphere were clear. Instead, the relatively shallow absorption feature suggests that there are clouds or haze suspended high in the planet’s atmosphere.
The cartoon in the right portion of the figure shows how this works: Panel (a) illustrates the passage of light emitted by a planet-hosting star through a clear planetary atmosphere, on the way to the telescope. As the light passes through, the water molecules (blue circles) imprint their spectral absorption feature. By contrast, panel (c) illustrates the passage for a cloudy atmosphere. The clouds block a lot of the light before it reaches the telescope, and so only the photons that pass high above the clouds, where there’s not much water to absorb light, get to the telescope. As a result, the water spectral feature is less deep.
The new techniques developed in Deming et al. (2013) may open the door to totally new astronomical observations. For example, the authors suggest that the significantly improved sensitivity of their technique may allow astronomers to directly observe meteorological variability (weather) on these distant exoplanets.
- July 16th, 2013
- Posted in Journal Club
- Comments Off on “Infrared Transmission Spectroscopy of Exoplanets HD209458 b and XO-1 using Hubble” — Deming+ (2013)
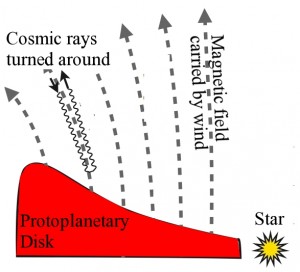
Figure 10 from Cleeves et al. (2013) showing the stellar wind driving cosmic rays away from the disk
In journal club this morning, we discussed a new paper by Cleeves, Adams, & Bergin that pointed out the important (and apparently previously unrecognized) role of stellar wind in the chemistry and dynamics of protoplanetary disks. Astronomers have long believed that cosmic rays induce significant ionization in protoplanetary gas disks around young stars. This ionization is important because (1) it drives chemical reactions, helping to set the initial compositions of planets, and (2) it causes the disk to interact with magnetic fields, producing turbulence in the disk, which affects where/how/when planets form. If, as suggested by the authors, the stellar wind can exclude cosmic rays from the inner reaches of the disk, then sufficient ionization to power these two effects requires some additional energy source. Cleeves and co. suggest decay of radio nuclides, which produces ionizing gamma radiation, may be able to take up the slack, but in our discussion, it was suggested that gamma rays might drive different chemistry from what was expected of cosmic rays.